Innovative Anti-Infectives
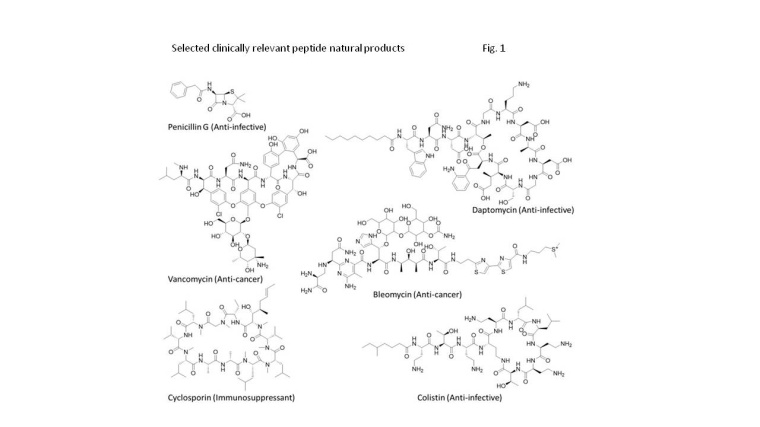
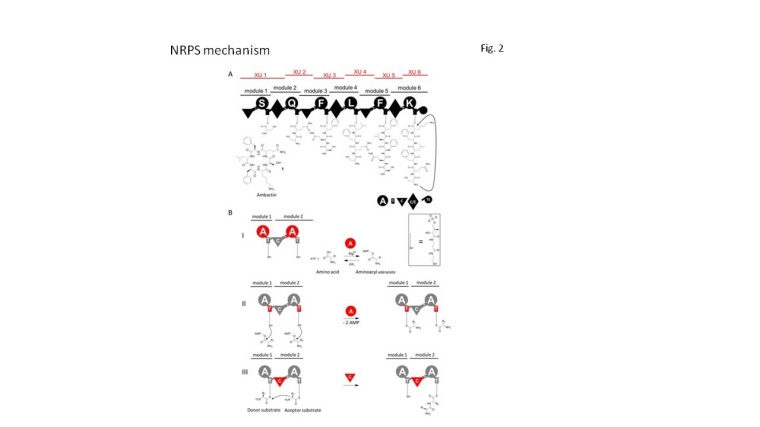
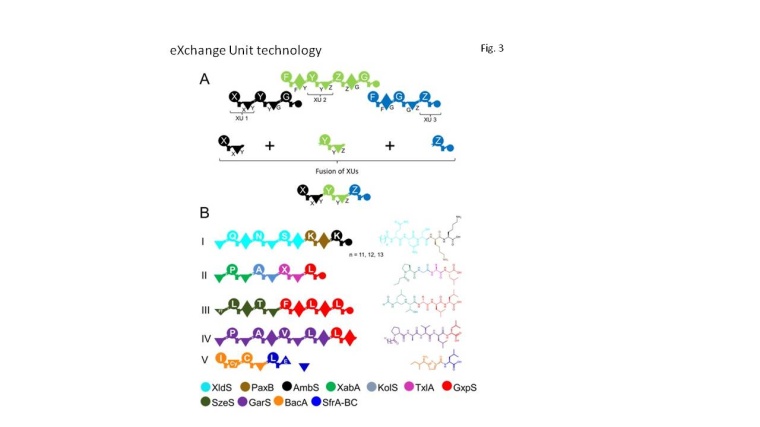
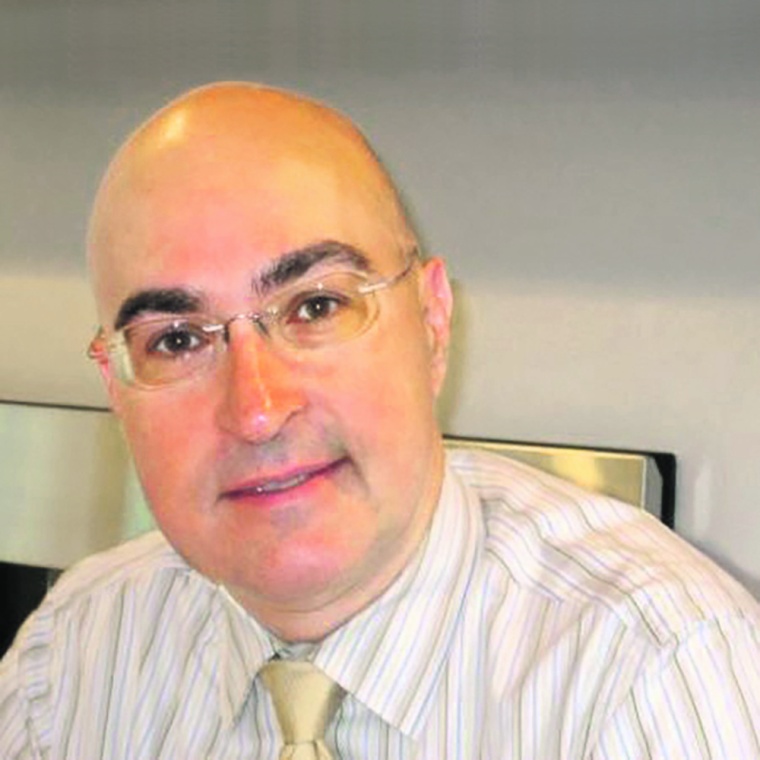
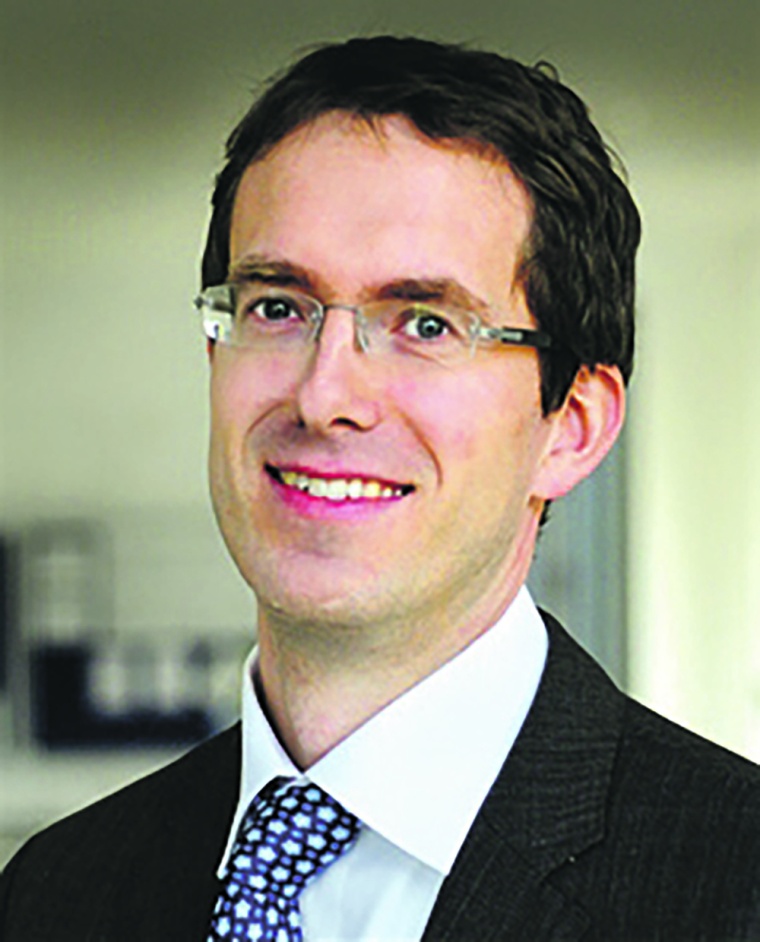
Natural products have been used since thousands of years for the treatment of a wide range of medical conditions in the form of traditional medicines. Today 60% of all new small molecule drugs are still derived from nature, and natural products have been the source or an inspiration for several approved drugs, e.g., for cancer treatment (taxol, epothilon), as immunosuppressant (ciclosporin) or anti-invectives (vancomycin, daptomycin) (fig. 1).
Today infectious diseases are still the second major cause of death worldwide with some 700,000 deaths. According to the World Health Organization (WHO), the world is facing a global public-health crisis as there is a growing risk of entering the pre-antibiotic era since more and more infections are caused by multi-drug-resistant bacteria. There are several reasons for the loss of antibiotics’ effectivity, both in wealthy nations and in poor. Economic, regulatory and scientific causes are further tightened by the increasing number of multidrug-resistant bacteria due to the misuse of antibiotics in clinics, agriculture, livestock breeding and feed industry. Diseases that were easy to control or had almost been eradicated due to the use of antibiotics have become or are soon becoming a threat again.
One source for new drugs with improved clinical properties, e.g., breaking antimicrobial resistance, is the chemical modification of existing drugs. To date, most clinically used natural product derivatives are created by means of semi-synthesis; a process where the original natural product is chemically modified after its isolation from a biological source. However, due to technical and chemical limitations, such modifications are mainly targeting the molecule’s periphery but leaving the backbone structure untouched.
Developing Novel Drugs Using Non-ribosomal Peptide Synthetases
One structurally highly diverse and pharmaceutically relevant class of bioactive drugs are peptide-based natural products. For instance, peptides have been identified with antibiotic, antiviral, anti-cancer, anti-inflammatory, immunosuppressant and surfactant activities. The market for peptide drugs is estimated around 10% of the entire pharmaceutical market and will increase in the future. In order to alter the backbone of these peptides, often bio-synthesized by non-ribosomal peptide synthetases (NRPS), the respective enzymatic machineries must be engineered. NRPS are very large and complex enzymes with a strict modular architecture (fig. 2). Each module consists of several catalytically active domains and is responsible for the activation, modification and elongation of the growing peptide chain. These non-ribosomal peptides are renowned for exhibiting unique structural elements, like D-amino acids, N-terminal attached fatty acids, N- and C-terminal methylated residues, heterocycles, glycosylated as well as phosphorylated amino acids and to date >450 different amino acids have been identified in these non-ribosomal peptides.
Very early on, the modular composition of NRPS raised expectations and desire for their reprogramming – realized for the first time in 1995 by the group of Mohamed Marahiel. Since then NRPS research dreams of using these enzymes like a molecular toolkit to modify bioactive peptides and create novel non-natural peptides in a tailormade way. During the last 20 years all NRPS core domains were biochemically characterized and crystal structures of domains and even whole modules were solved. Yet, to date most attempts to achieve NRPS re-engineering have yielded biosynthetic machineries that are either greatly impaired in their activity (0.1-0.5 % compared to wildtype production titers) or that are completely non-functional.
The same applies even for the currently most successful domain substitution campaign, reported by researchers at Cubist Pharmaceuticals (Lexington, USA), in the daptomycin (Cubicin) producing NRPS of Streptomyces roseosporus. Most of the re-engineered NRPS assembly lines were impaired, resulting in reduced titers of the desired peptides. Moreover, the campaign failed to introduce generally applicable and reproducible guidelines that may be transferred to other systems.
In the 2010s, the fundamental biochemical issues of NRPS were solved and recent advances made in the areas of crystallography and cryo-electron microscopy (cryo-EM) delivered new insights on the dynamics, mechanics and domain-domain interactions of NRPS and other megasynthases like polyketide synthases. In brief, it became clear that NRPS are only functional if, besides preserving the catalytic activity of individual domains, domain-domain interactions are maintained, too.
First structure-based strategies maintaining interfaces of NRPS domains were published by the groups of Piel (2013) and Hilvert (2015). Both groups successfully exchanged the active site forming amino acids of adenylation domains to alter their substrate specificities resulting in the production of new peptide derivatives. Another very recent strategy, published by Meyer, Süssmuth and colleagues (2017), enabled the biosynthesis of enniatin and beauvericin derivatives by combining structurally and biochemically highly related NRPS. The new peptide derivatives showed up to 12x increased activity against Leishmania donovani and Trypanosoma cruzi compared to the reference drugs miltefosine (Miltex) and benznidazole (Rochagan), respectively. Noteworthy, besides the very good bioactivity of the new derivatives, it had been possible for the first time to produce new-to-nature peptides in an industrial relevant scale (1.3 g L-1).
Generating Novel Non-ribosomal Peptide Synthetases
All strategies introduced so far are based on re-programming certain NRPS to alter single amino acid positions of naturally available peptides to create new derivatives. Moreover, all reported and successfully applied strategies lack transferability and applicability to other systems. Consequently, a reliable, stable, fast and cost-effective technology platform enabling the de novo design of any desired peptide from scratch has been lacking completely up to now.
In 2012, a research project funded by the Federal Ministry of Education and Research (BMBF) in Germany dedicated to close the lack of knowledge, has started to develop new and reproducible strategies for both reprogramming naturally available NRPS templates and designing novel “synthetic” NRPS machineries. To face the complex task, a structure-based and mechanistic in silico approach was chosen; all publicly available NRPS crystal structures were analyzed bioinformatically to identify feasible recombination rules. After a five-year research phase, a first technology platform enabling the biotechnological production of tailormade new-to-nature peptides was established that is backed-up by several patents. The eXchange Unit (XU) technology provides, for the first time, both reproducible and explicit guidelines for the design, cloning and biosynthetic production of non-ribosomal peptides of desired length, composition, modification and configuration. While considering the identified guidelines as well as applying non-natural domains for termination and regeneration naturally available peptides, peptide derivatives, and new-to-nature linear-, cyclic-, and lipo-peptides were produced by recombining up to five XUs from four different organisms with good yields (fig. 3).
Outlook
Due to the very urgent need to develop new innovative anti-infectives and general efforts to reduce the use of petrochemical precursors, reagents, and solvents, synthetic biology as well as the biotechnological production of peptides is highly attractive. Recent advances in the field of combinatorial biology will attract and inspire other scientists to develop solutions that extend the boundaries of what is currently possible. In the near future, progress made in the areas of crystallography, electron-microscopy, genome mining, editing, and gene synthesis, machine learning as well as 3D printing will enable the in silico calculation of novel biosynthetic gene clusters. These will then be synthesized (or even 3D printed) and finally heterologously expressed in an industrial super host to produce the desired tailormade drug.
References are available from the authors.